|
 |
Research Projects |
Over the past eighteen years
I have developed an interdisciplinary undergraduate and graduate
research program in Applied and Computational Mathematics. The
work has an emphasis on combining dynamical systems theory, group
theoretical methods, i.e., equivariant bifurcation theory, and
computational methods to model, analyze, and predict the behavior
of Complex Nonlinear Systems.
At the beginning of my
career, I was interested in pattern formation and worked on PDEs
and ODEs to study, numerically and analytically, the symmetry-breaking
bifurcations that give rise to cellular flame patterns. I was also
interested in Mathematical Biology and worked with Prof.
Marty Golubitsky on the existence and stability of heteroclinic
connections in coupled cell systems. I then extended that work
to study the rhythms of the heart beat of medicinal leeches. In
the 2000's, my work focused on applications to engineering and
physics with the fundamental principle to mimic what nature does
best: to create networks of tightly interconnected devices with
collective optimal responses. In collaboration with engineers and
experimentalists, we worked on deterministic and stochastic models
of: biologically-inspired networks of magnetic and electric field
sensors; 2D arrays of superconducting quantum loops; networks of
vibratory gyroscopes; and arrays of nano-oscillators. In the past
few years, previous works have led us into new topics: networks
of energy harvesting systems; networks of Hamiltonian cells; computational
methods to simulate and characterize Ozone concentrations and related
pollutants from PDEs; and analysis of physiological measurements.
Below are some representative examples of previous and current projects.
|
Biologically-inspired
Sensor Devices
A major theme of my research is
to explore the advantages exhibited by biological network
systems to model, design, and fabricate, sensory devices
that can harness cooperative behavior to increase sensitivity
at low power. For instance, sharks can sense extremely
weak electric fields due to the collective output of
thousands of detectors called ampullae of Lorenzini.
A general question is to investigate what aspects of
the network dynamics are due to network topology and
what aspects are due to details of the internal dynamics
of each cell. In collaboration with scientists and engineers
from the Space and Naval Warfare Center, San Diego, we
have modeled, analyzed and fabricated a new generation
of highly-sensitive (up to 400 times more sensitive than
conventional sensors) networks of magnetic and electric
field sensors. The network exploits the bistability
of each unit and the topology of heteroclinic cycles
induced by cyclic symmetry. The work has been supported
by NSF, ONR, DoD, and has lead to internships for participating
students, manuscripts, and multiple U.S. patents, please
see vitae. We are currently developing the concept of
a Universal Ultra Sensitive Network System capable of
detecting: magnetic field, electric field, seismic, acoustic,
infrared, thermal neutrons, chemical and biological multi-agent
sensing (CMAS), and other signals in one single unit. |
|
|
MEMS Gyroscopes
Funded
by the National Science Foundation, we have shown, analytically
and computationally, proof of concept that networks of coupled
gyroscopes, modeled by
can offer significant performance improvements by reducing
the negative effects of noise while minimizing phase drift.
We also developed a novel scheme, a drive-free network-based
gyroscope system, in which the coupling topology can lead
to self-regulated limit cycle oscillations in the drive-
and sense-axes with stable constant amplitude and phase-locking.
This work was selected and advertised by AIP (American Institute
of Physics) as a research highlight due to its originality
and novelty of the concept. The next step is to explore a
possible MEMS realization of the models. In addition, the
work has led to many unanswered questions regarding the nature
of the bifurcations in large networks. A perturbation analysis
is not well suited, so we are currently investigating a different
approach via coupled Hamiltonian systems. |
|
|
|
Energy Harvesting
Energy harvesting devices are key to a wide range of technologies: wireless sensor networks, micro wind turbine, thermoelectric generators, health monitoring systems, human-generated power devices for biomedical applications and, of course, consumer products such as automatic wristwatches and TV remote controls, to the cloud. A major challenge to advance these technologies is the fact that the amount of electrical energy produced is small for these devices. To circumvent this problem, several groups have considered nonlinear materials and have proposed innovative designs and techniques, which together can improve power output. The improvements are, however, reaching the fundamental limits of power output that can be harvested with single units. Only in recent years networks of multiple harvesters have started to attract attention but a comprehensive analysis of the governing equations has not been carried out yet. This is a more recent project in which I am currently modeling and analyzing the power output response of a network of energy harvesters made up of magnetorestrictive materials. One of the goals is to determine scaling laws for the energy output as a function of the size of the network and the topology of connections.
|
|
|
Microwave Signal Generator
The 2007 Nobel prize in Physics was awarded jointly to
Albert Fert and Peter Grunberg for their discovery of
the Giant Magnetoresistance Effect (GMR). The GMR effect
can convert the magnetic precession of an electron into
microwave voltage signals and turn the oscillator into
a Spin Torque Nano-Oscillator (STNO) but its power output,
about 1 nW, is too small for practical applications.
A possible solution is to synchronize several STNOs so
that a coherent signal with a common frequency and phase
can be extracted from the ensemble to produce a more
powerful microwave signal. Supported by NSF, I am
currently studying and classifying the various coherent
statesthat an ensemble of STNOs can produce, finding
conditions for the existence and stability of such coherent
states, determining the effects of different couplings
and connection topologies.
|
|
|
Networks of Superconducting Loops
Supported by NSA Tactical SIGINT Technology Program, we have conducted extensive analysis and computer simulations of the collective voltage response of networks of thousands of superconducting quantum loops. The aim is the development of a sensitive, low noise, significantly lower Size, Weight and Power (SWaP) antenna, capable of meeting all requirements for certain class ships for Information Operations/Signals Intelligence (IO/SIGINT) applications in Very High Frequency/Ultra High Frequency (V/UHF) bands. The device will increase the listening capability of receivers by moving technology into a new regime of energy detection allowing wider band, smaller sized, more sensitive, more stealth systems. The smaller size and greater sensitivity will allow for ships to be de-cluttered of their current large dishes and devices. Ongoing work includes derivation of asymptotic analytical approximations to the exact solutions. Such solutions could be used to expedite future analysis of other array configurations.
|
|
Computational Models of Ozone Formation
Tropospheric ozone is deleterious to both human and ecosystem health. It is produced through non-linear chemical reactions involving precursor emissions of nitrogen oxides (NO_x) and volatile organic compounds. Comprehensive air pollution models include detailed treatment of the emissions, transport (advective, turbulent, clouds), and chemistry dictating the spatial and temporal distribution of O_3 and related precursor species. From a policy standpoint a question of interest is to determine how much an individual source contribute to the O_3 at a given location and time. While many techniques ranging from simple brute-force methods to formal sensitivity analysis have been used, the ``backward'' attribution of O_3 at a given location/time to its sources remains challenging due to the non-linearities in the system. In this project we will address the question of determining the source of O_3 emission by solving an inverse problem from a Partial Differential Equation model for ozone concentration.
|
|
Basins of Attraction
We have developed computational methods to visualize basins
of attractions for coupled bistable systems. Code is available
under this folder: CCFM_Manifolds.
|
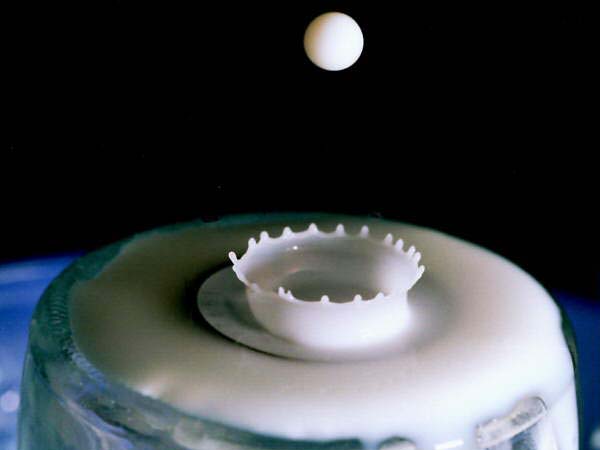 |